In the context of a transition to less carbon-intensive energy systems, hydropower is the most important because it is renewable, flexible, storable and controllable. But beyond the specificities that distinguish it from other forms of electricity production, it is also the very great diversity of developments that characterizes hydropower.
What do they have in common,
- between a small hydroelectric power plant built on a natural or artificial weir in a river, with a capacity of a few hundred kW, and the Three Gorges Dam power plant in China, with a capacity of 22,500 MW, the equivalent of five nuclear power plants,
- or between the La Bâthie power plant in the Beaufortin region, producing 1,100 GWh/year for an installed capacity of 540 MW, and the Beauchastel power plant on the Rhône, whose production of 1,200 GWh/year is slightly higher with an installed capacity of 340 MW?
What these developments have in common is the transformation of the potential energy of water into electrical energy according to the formula: P = ρgQHη, where:
- P (W) is the power,
- ρ (rhô) (kg.m-3) is the density of water,
- g (m.s-2) is the acceleration of gravity at the location considered,
- Q (m3.s-1) is the volume flow,
- H (m) is the head and η (eta) is the efficiency [1] (Read : Hydroélectricité : les conduites forcées de l’entreprise Bouchayer-Viallet à Grenoble).
Beyond this common point, all these facilities are specific to the site on which they are built, the structures, their equipment have been specially studied. Their modes of operation, their role in the management of a network, their effects on the environment, are also very specific to each facility. But all in all, the appeal of hydropower lies in its renewable, flexible, controllable and storable nature, to which are added a high rate of return and a long life span.
1. The great diversity of developments
The great diversity of hydropower is expressed in the wide range of power outputs of the plants, the diversity of arrangements of the plants according to their location and the hydrological characteristics of the river that feeds them, as well as the role that they play in the management of the network to which they are connected.
1.1. A very wide range of power
Hydroelectric generating units, i.e. the turbine and the generator, cover a range of capacities from a few kW to 1000 MW for the most powerful Francis-type turbines. The generating facilities, i.e. the powerhouse and its equipment, range from a few kW to 22,500 MW for the most powerful powerhouse currently in operation, at the Three Gorges Dam site in China (Figure 1).
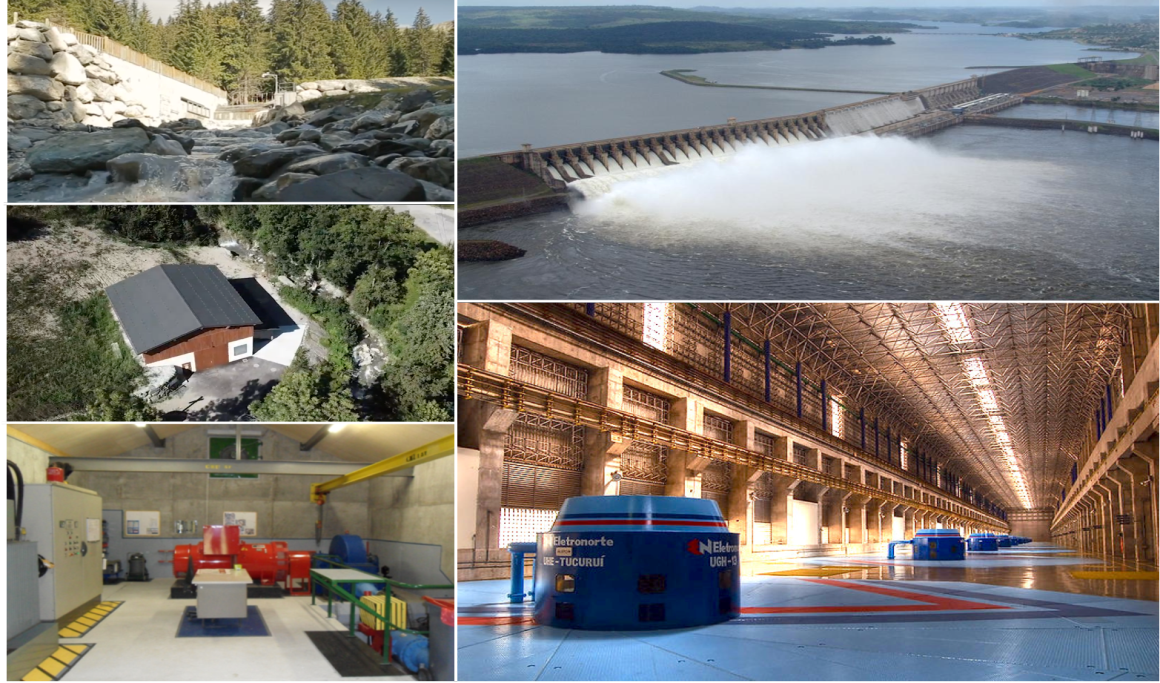
Fig. 1. Bozel (1,7 MW) and Tucurui (8,3 MW) power plants. [Source : Photos GEG, Zeco, Antonio Castro, Nilton Ramos]
In 2020, of the ten most powerful power plants in operation in the world, nine are hydroelectric plants: Three Gorges in China (22.5 GW), Itaipu in Brazil (14.0), Xiluodu in China (13.9), Guri in Venezuela (10.2), Tucurui in Brazil (8.4), Grand Coulee in the United States (6.8), Xiangjiaba in China (6.5), Longtan in China (6.4), Saiano-Shushensk in Russia (6.4).
Because of this wide range of power, and although there is no official definition, it has become customary to distinguish between small hydropower and large hydropower. This boundary is sometimes used in regulations to support the development of renewable energies, with only small hydro being considered. It varies from one country to another, from 10 MW in France or Norway, to 50 MW in China or Canada. For turbine manufacturers, small hydro belongs to the field of standardized machines (Read: La petite hydroélectricité en France).
Within small hydro, we distinguish:
- the pico hydro, generally relative to installations of less than 5 kW (Read: L’hydraulique villageoise dans les pays en développement),
- micro-hydro to those of less than 100 kW,
- mini hydro below 1 MW.
1.2. A large range of heads
The diversity of the waterfalls that can be equipped leads to very different types of structures. Hence the unofficial nomenclature :
- high heads, over 200 metres,
- medium heads, between 40 and 200 metres,
- low heads, below 40 metres.
The world record for head is held by the Bieudron power station in Switzerland, with a head of 1,883 m. In France, the record is held by the Portillon power station in Haute-Garonne (1,420 m).
Harnessing a waterfall can be done directly on the site of the waterfall, by installing the power plant directly on a river weir or at the toe of the dam for medium head facilities or in an “in-line” arrangement for low head facilities, as opposed to “bypass” facilities (Figure 2). In this case, a portion of the natural watercourse is short-circuited, in which it is necessary to maintain a minimum flow.
In the case of high head schemes, a set of intake structures (water intake, free surface canals, pressure galleries, lined shafts or penstocks) makes it possible to conduct the water from the reservoir to the power plant, then from the power plant to the place of restitution in the watercourse. Very often, all these structures, including the power station, are underground (Read: Hydroélectricité : les conduites forcées de l’entreprise Bouchayer-Viallet à Grenoble). High-head schemes often include a complex network of galleries and intakes to collect and pool water from several catchment areas for turbining in a single power station (Figure 3).
1.3. Different types of operation
Several types of operation can be distinguished according to the capacity of the reservoir.
a/ Developments where the capacity of the reservoir is low in relation to the equipment capacity (discharge) and the average flow of the watercourse. These are typically those whose reservoir filling time is less than two hours at the average flow of the watercourse. They are called run-of-river. This type of facility must turbinate the entire incoming flow, with very little or no modulation capacity. This is the case of the facilities built on large rivers such as the Rhône (Read: Les grands aménagements hydroélectriques : Pierre-Bénite et les groupes bulbes) or the Rhine, in France.
b/ Developments where the capacity of the reservoir is such that the filling time remains less than 15 days at the average river flow. This type of facility allows daily or weekly hydropeaking (Read: Les grands aménagements hydroélectriques : Génissiat (I) and Les grands aménagements hydroélectriques : Génissiat (II)).
c/ Developments where the capacity of the reservoir is such that the filling time is greater than 15 days at the average river flow. They are intended for peak production.
d/ Pumped storage power stations (PSP). These facilities (Read: Les stations de pompage (STEP)) have two reservoirs at different altitudes and are designed to store (by pumping from the lower reservoir to the upper one) the energy produced during periods when production is higher than consumption and to inject it into the network by turbining during periods of high consumption (Figure 4).
e/ Tidal power plants. In 2020, there are only two tidal power plants with significant power: in France, La Rance, 240 MW (Figure 5) and in South Korea, Shiwa, 254 MW. However, projects exist, either in natural sites or in artificial lagoons, notably in the United Kingdom[2]
2. Unique projects
Because of the specific characteristics of each site, a hydroelectric facility is always a specific project, unique in all its components (Read: Les ouvrages hydrauliques).
- A dam is designed specifically for a particular site. The choice of the type of dam itself depends on the geological, topographical (shape of the valley) and seismic characteristics of the site, on the hydrology (flood characteristics) as well as on the resources that may be available in the vicinity (aggregates for concrete, rockfill, clays).
- The routing and characteristics of the water supply structures to the power plant and of the tailrace channels (free surface channels, tunnels and pressure galleries, surge tanks, penstocks and lined shafts) are also a function of the topography and geology of the site.
- The power plant itself, whether underground or in the open air, is designed according to the equipment it is to house.
Moreover, unlike other electricity production facilities, for which the production equipment is standardised (steam turbines, gas turbines, nuclear power plants, wind turbines, photovoltaic panels), the equipment of a hydropower plant (turbines and generators) is also specifically designed for each project[3]. This specificity is due to the fact that the determining element is the turbine, which transforms hydraulic energy into mechanical energy, which is then transmitted to the generator, which transforms this mechanical energy into electrical energy. The sizing of the turbine is the result of an economic optimum according to the following reasoning.
- The first step is to determine the flow rate of the power plant, i.e. the maximum discharge that can be turbined. For a reservoir facility, intended for peak production, this discharge is determined on the basis of the annual volume accumulated and the number of operating hours desired (economically valued hours). For a run-of-river facility, the equipment discharge is a proportion of the average annual flow of the river. The higher the design discharge, the more power can be used for high flows, but this means that the power plant will only operate at maximum power for a short period of time. The equipment discharge is therefore the result of an economic optimum beyond which the additional cost of increasing capacity is not recovered by the corresponding production.
- Once the equipment discharge is known, the number of production units must be determined. This number will depend on the variability of the flows and the capacity of the turbines selected to operate over wide ranges of discharge, but also on the technological capacities, as there are maximum dimensions and power for any type of turbine. The possibility of carrying out maintenance while limiting production losses will also be taken into account.
- Once these operations have been carried out, the basic values for turbine sizing, head and discharge, are defined. In combination with other more specific criteria (cavitation, water loaded with abrasive materials), experience shows that only in exceptional cases are identical characteristics found for two different power plants.
- The dimensioning of the turbine leads to determine, among other things, its speed of rotation. This rotation speed and the maximum power are the two basic parameters for the sizing of the generator. Here again, only in exceptional cases are identical characteristics found for two different power plants.
The efficiency of a turbine varies according to the head, which changes according to the level in the reservoir, for example, and the discharge. The weighted average efficiency is obtained by determining the operating time for each operating point (head-discharge pair). The maximization of this weighted average efficiency is obtained by optimizing the hydraulic profile of the turbine, shape of the blades or vanes (Figure 6) by means of numerical modelling called Computational Fluid Dynamics (CFD) and physical modelling, performed for each project (Figure 7).
For high-powered machines, this optimization of efficiency is of considerable economic importance and warrants specific studies (Table 1).
Table 1. Optimization of the weighted average efficiency
These specificities result in a high cost and duration of the design phase. These studies also call on a large number of disciplines: hydrology, geology, hydrogeology, hydraulics, topography, geotechnics, hydro-biology, environmental sciences, mechanics, electricity, computer science, among others.
However, in the field of small hydro, given the power involved, cost reduction is a crucial issue for project profitability. In this context, more attention is paid to reducing the cost of design studies by standardizing equipment to the detriment of the absolute level of performance. For example, the cost of a model test is the same regardless of the power of the industrial machine; it will never be profitable on a low power unit.
Because of the very different nature and scale of hydroelectric projects, construction costs vary greatly from one project to another. Civil engineering costs are site-specific, depending on the characteristics of the project (volume of concrete, length of water courses, geology) and the availability of materials near the site. The cost of equipment, supplied by international manufacturers, is essentially a function of the economic situation in the sector and the origin of the equipment (European versus Chinese manufacturers, for example).
Generally speaking, for the same installed capacity, high head projects, which use lower flows, are less expensive than low head projects. Similarly, high capacity projects generally have lower construction costs per MW installed. This results in a large variability in capital costs and the Levelized Cost Of Energy (LCOE) (Table 2).
Table 2. Costs of hydropower. [Source: REN 21 Renewables 2018 report]
The insertion of each development in its societal and natural environment is another consequence of its specificity. Harnessing a natural weir in a river will have almost no effect, neither on fish migration, nor on the sediment transport. It will not modify the hydrological regime of the river. On the other hand, a more important development, with the creation of a large reservoir, will have significant social and environmental consequences (Read: Hydroelectricity: environmental and societal impacts).
The same applies to the effects on land use planning, depending on the size of the project, which may affect several kilometres, or even tens or even hundreds of kilometres. Communication routes are removed, diverted or created. Other uses of water are associated with the production of electricity: irrigation, navigation, recreation, low-water support, etc. Populations and human activities may be disturbed or relocated.
In this sense, a hydropower development is not a simple industrial installation, it is a real land use project which must be studied as such, with the participation of all the concerned stakeholders.
3. The qualities common to all hydropower schemes
Beyond the diversity of the facilities, hydropower draws its attraction from a certain number of specificities.
3.1. A renewable, controllable, flexible and storable resource
The management of an electrical network requires a permanent balance between production and demand. Insofar as the latter is not, or not yet very, controllable, it is essential to have sources of production capable of adjusting permanently to its variations (Read: Stockage hydraulique : atouts et contraintes and Hybridation des systèmes électriques : les atouts de l’hydroélectricité).
The integration, in strong growth, of intermittent means of production, including wind and solar photovoltaic, whose production variations are sometimes brutal and in opposition with the variations of the demand, increases the need for flexibility of other means of production.
Hydropower is the most suitable means to meet these needs, both because of its flexibility [4] and its storage capacity [5], taking into account the response time values of the different means of electricity production (Table 3).
Table 3. Pick-up/drop-off speed in % of rated power per minute.
The start-up time of a hydropower unit is very short, on the order of a few minutes. The most modern PSPs also have the capacity to change their operating mode from pumping to turbining and vice versa in a few minutes, or even less than a minute for plants equipped with ternary groups with hydraulic short-circuiting. Hydropower, including PSPs, thus has a key role in matching generation to electricity demand (Figure 8).
These performances also allow hydropower to play an identical role in the provision of ancillary services that ensure the quality of the energy delivered to consumers in terms of frequency stability and voltage level. While this is primarily the case for reservoir facilities and PSPs, it is also the case for run-of-river facilities, which can also react quickly when needed. During the blackout of November 4, 2006 in Europe, the Rhone river facilities provided an additional 270 MW of power in 2 minutes. Some hydroelectric power plants are also capable of starting up without an external energy source, i.e. in black start, and of carrying out voltage recovery, which is necessary to rebuild the network in the event of a blackout.
3.2. High rate of return
The energy rate of return (ERR) is the ratio of the energy supplied over the total lifetime of the plant to the energy consumed in its construction, operation, maintenance, decommissioning and fuel supply. Hydroelectricity is the most efficient of the various electricity generation technologies in terms of this criterion (Table 4).
Table 4: ERR of different energy sources. [Source: Di Ugo Bardi (2005). Il ritorno energetico sull’investimento energetico. www.aspoitalia.net]
3.3. Long service life
Compared to other power generation facilities, hydropower facilities have exceptionally long lives and operating lives (Figure 9). Those built in the first half of the 20th century are still in operation and will remain so for many years. Civil engineering structures, properly monitored and maintained, can last well over a century. The equipment also has a lifespan of several decades and their modernization, or even replacement, allows the continued operation of the facility while improving its performance and reducing maintenance costs (Read: Hydroélectricité : l’opportunité de la réhabilitation des centrales).
Fig. 9. Lifetime of the main equipment
A well thought-out modernization allows to adapt the plant to possible evolutions of the context such as a variation of the hydrology due to the climate change, legislative and regulatory evolutions or a change of the exploitation mode due to the integration of intermittent sources. It is also an opportunity to improve its environmental integration with interventions such as the construction of fishways, the adoption of fish-friendly turbines or oil-free technology.
As an example, the Chancy-Pougny plant, commissioned in 1924, has been the subject of a vast modernisation programme allowing an increase in the average annual production from 210 to 255 GWh, the construction of a fish pass, the reinforcement of the dam with regard to the risk of earthquakes, the automation and remote control of the plant (Figure 10).
Notes and references
[1] The article does not deal with tidal turbines, marine or river, which exploit the kinetic energy of currents (See: Tidal turbines and River tidal turbines).
[2] http://www.tidallagoonpower.com
[3] When talking about the industrial turbine, a turbine manufacturer talks about a prototype (as opposed to the model turbine used for the development and validation of performance)
[4] The European XFlex Hydro programme (https://xflexhydro.net) aims to demonstrate all the possibilities offered by hydroelectric installations to support the development of intermittent energy sources.
[5] STEPs account for approximately 96 to 97% of all energy storage, all technologies combined (source: www.ren21.net).
————————————————–
The Encyclopedia of Energy is published by the Association des encyclopédies de l’environnement et de l’énergie (A3E)(www.a3e.fr), contractually linked to Grenoble Alpes University and Grenoble INP and sponsored by the Academy of Sciences.
To cite this article, please mention the author’s name, the title of the article and its URL on the Encyclopedia of Energy website.
The articles in the Encyclopedia of Energy are licensed under the terms of the Creative Commons Attribution-Noncommercial-No Derivative Works 4.0 International License.