The rise of variable renewable energies (VRE) encourages the development of new intersectoral couplings Power to Gas and Power to Heat . Their potential development encounters many obstacles because the very capital-intensive techniques they use are only profitable with a high rate of use which depends on the length of periods of low electricity prices in electrical systems with high shares. from EnRi. Overcoming these obstacles requires strong energy governance.
The focus of low-carbon transition policies on intermittent renewable energies (RE) in the electricity sector leads to the search for economic means to cope with the variability of these productions and to give a value to the surplus of RE electricity production compared to the hourly demand in systems where the share of these sources is high. At the same time, the desire to replace natural gas by green gas in the gas system should lead to building bridges between electricity production and the gas system with the production of hydrogen by electrolysis, which would be extended by the production of synthetic natural gas (SNG) injected into the gas system and used to power different types of vehicles.
There are even proposals to re-generate electricity from this SNG when the time is right, with this inter-seasonal diversion serving as storage. This could lead to the development of Power-to-Gas (P2G), on the one hand, and to the development of a hydrogen economy for various industrial, transportation and gas system uses, on the other. At the same time, the uses of green electricity in industrial heat uses and space heating through district heating via large heat pumps that act as Power-to-Heat (P2H) gateways, are far from being forgotten in these integrative approaches.
Electrification can thus also be used to decarbonise part of the demand for passenger transport by light vehicles with the development of electric vehicles in countries where electricity production is largely decarbonised. For the electric sector, the different types of couplings are sources of flexibility to absorb fluctuations in the production of electric EnRi. The electrification of uses, combined with digitisation, opens up many possibilities to reliably link power generation with energy demand in real time and at different levels of the power system, in transport, buildings and industry, at the points of location of different types of consumers [1]
1. In search of moving beyond sectoral approaches
Low-carbon energy transition approaches, defined on a sector-by-sector basis, are bound to be outdated. The news differs from the initial studies on the development of large-scale EnRi in the electricity sector, which only took into account single-service technologies; they only extended prospective thinking to electrical storage techniques and to the potential opened up by smart grids
for demand management and the integration of self-producers. Now, we are considering deploying technological sectors straddling several sectors: production of hydrogen and synthetic methane from the electricity surplus from the production of electric EnRi, or heat production using large heat pumps.
On the strength of the market paradigm that dominates the designs of energy-climate policies in the European Union (EU), the protagonists of these intersectoral couplings claim that intertwining the sectors in transition policies by integrating electricity, gas and heating networks would make it possible to limit the costs to society. This solution would be more economical than optimising the subsystems only separately, as it would allow synergies to be exploited by promoting intelligent conversions from one energy carrier to another.
Such ideas are being assiduously explored in countries where, for reasons of nuclear waste andCO2 capture and storage (CCS), only EnRi can be used to achieve carbon neutrality in the electricity sector (Read: Electricity storage). This requirement is due to the intermittent nature of wind and solar production, which must find palliatives to ensure hourly, daily and weekly balance through the development of new sources of flexibility. Likewise, solutions must be found to use the surplus of EnRi’s production in relation to hourly demands, more and more numerous as they are deployed, in preference to the erasing of part of the wind or solar PV production. One use of the production surpluses could be to produce green hydrogen by electrolysis, as part of the greening policies of the gas system in search of carbon neutrality (Read: “Green” hydrogen production).
These ideas are very much present in the rhetoric of the European Commission (EC) on energy transition and in the rhetoric of the National Energy Agencies of the leading countries in the promotion of electric EnRi (Figure 1). These approaches are intended to be integrative by advocating the large-scale implementation of different types of cross-sectoral coupling, and by demonstrating their economic interest using prospective scenarios (Read: European Union: Climate and Energy 2030).
Such linkages are not self-evident, however, for reasons related to the economic characteristics of the technologies they mobilize and the institutions shaped by the principles of competition. Before examining the obstacles to be overcome and the paths to be explored to achieve this (Read: The cross-sectoral “Power to Gas” and “Power to Heat” couplings: what role in the energy transition?( Part 2)), it is appropriate to consider the following questions:
- the different ways of electrification of uses, then the different channels of Power-to-Heat (P2H) and Power-to-Gas (P2G)[2];
- the electrification of heat uses, via heat pumps in industry and district heating compared to established techniques, conventional boilers and cogeneration, some of which are powered by RE (biomass or geothermal energy, among others) ;
- modes of production of hydrogen and synthetic methane with those of other green gases, whose development challenges are considerable;
- the chances of competitiveness of the P2H and P2G coupling options, identifying the main constraints and regulatory obstacles encountered along the way.
2. Electricity-heat couplings in the decarbonation of uses-heat
Energy consumption in heat-refrigeration uses is one of the main end uses of energy in the EU (Read: Energy in the European Union 2000-2014). As such, it is responsible for a significant share of CO2 emissions in industrialised countries. In 2015, more than a quarter of energy demand was used for space heating and about a sixth in the form of industrial heat. It therefore represents an important issue of decarbonisation through the direct or indirect use of renewable energy that can be obtained:
- the end use of electricity by heat pumps or electric boilers ;
- the transversal integration of vectors through combined heat and power production based on biomass for large buildings and district heating; indeed, biomass remains the most widespread renewable heating source in Europe for individual, collective and network heating (Read Biomass and energy) ;
- in district heating and the heating of large tertiary buildings (Read District heating networks), the integration of the vectors through their co-production from RE is also possible with cogeneration installations powered by biogas or biomethane (gas turbines, fuel cells, heat engines, among others) or by means of gas heat pumps operating with these same green gases;
- space heating.
In the field of space heating, district heating networks have the greatest potential for the development of P2H coupling as an indirect mode of decarbonation, alongside the direct use of RE (biomass, geothermal) or urban waste. Indeed, P2H allows an indirect use of electric RE, via the conversion into heat of the surplus MWh produced during periods of low hourly prices on the electricity market, conversion carried out by electric resistance boilers, or much better, by high-performance heat pumps[3]. The latter have a high coefficient of performance (COP) by providing about three times more thermal energy than their electricity consumption, while other technologies, such as electric boilers or direct electric heating, have an efficiency of slightly less than one (Figure 2).
The stock of heat pumps in Europe reached almost 10 million units in 2016, which is low compared to the European building stock. Heat pump sales stagnated between 2008 and 2015 at around 800,000 units per year[4]. A voluntarist policy aiming at the large-scale deployment of heat pumps in individual or collective heating of housing is quite possible, as shown by the case of Sweden which, in the 1990s, set up support mechanisms for the adoption of heat pumps[5]. In this country, from 1990 to 2014, fossil fuels in individual and collective heating have been replaced by both decarbonated, hydraulic + nuclear electricity, thanks to direct electric heating and individual heat pumps, but also by heat from district heating increasingly supplied by biomass. Both sources now meet up to 75% of heating demand for buildings, with more than 50% of this heat coming from district heating, compared to around 12% in the EU as a whole in 2017.
2.2. heat in industry
In the industry, the development of the indirect use of RE will take place in two ways, depending on the temperature level of the use in question. For low-temperature (LV) and medium-temperature (MT) uses, the industrial heat demand could be partly electrified with the use of large heat pumps. On the other hand, the high temperature (HT) heat demand must be dealt with by another indirect way, i.e. the use of hydrogen that would come from electrolysers fed by the surplus production of electric RE[6].
For MV and LV uses, the choice of industrialists will obviously be made on the basis of the competitiveness of the heat pump solution, which will depend on the number of hours during which electricity on the market is purchased at low cost, i.e. 15-20€/MWh instead of 50-60€/MWh on average, which will depend on the share of RE production in the total production. This solution may receive an additional boost if governments manage to put in place a high carbon price,
- via the European permit system for large emitters, in particular by setting a high floor price,
- or a carbon tax for small industrial consumers, which would increase the cost of heat from fossil fuel boilers.
2.3. indirect coupling of electric RE and district heating supply
While district heating (Read: District heating networks) meets on average 12% of European heat demand, it accounts for a very large share of the heat demand of buildings in some countries such as Denmark (62% in 2017), Sweden (52%) and Poland (43%). Germany has the largest number of district heating networks in Europe (1,342 in 2014) and the largest supply in terms of size (70 TWh thermal), but this sector weighs much less in the supply of heat to dwellings (8%) given the size of the market (Table 1).
Biomass is the most common form of renewable energy source used in district heating. Its use is increasing in the networks of several European countries, and some technologies are already competitive with fossil fuel boilers in some countries (Read: From the discovery of fire to biomass combustion). Regarding the use of geothermal energy, more than 240 heating networks in Europe are powered by this energy (Read : Geothermal energy). The first were developed in countries with a significant hydrothermal potential (France, Germany, Iceland, Italy). But over the last 15 years or so, heat pumps based on geothermal energy have enabled less well-endowed countries to develop heat networks in order to green their energy mix (Netherlands, United Kingdom). As a result, the dynamic development of the P2H intersectoral coupling should increase this use of geothermal energy.
Table 1: Share of district heating in some European countries in 2017
Germany | Denmark | Sweden | Poland | Finland | Austria | France | EU | |
Share in domestic/tertiary heat | 9% | 62% | 52% | 43% | 38% | 8% | 3% * | 12% |
Energy delivered (TWh) | 70 | 29 | 48 | 60 | 30 | 20 | 10** | nd |
Number of networks | 1342 | 394 | 250 | nd | nd | nd | 550 | nd |
Notes : *The share of district heating in Italy, the Netherlands and the United Kingdom is also at the 3% level.
**The share of RE in the supply of district heating in Franc in 2017 is 56%.
[Source: © Euroheat&Power, Country by Country 2017]
Among all the countries, it is in Denmark that the most innovative inter-sector coupling solutions are starting to be tested, as it is the country where district heating is the most developed (62% of the heat needs of homes) and where the electricity policy based on intermittent RE is the most active (47% wind power in 2018). It is therefore in this country that large heat pumps and electric boilers are being considered as heat sources for district heating networks alongside biomass for boilers and cogeneration, in order to take advantage of the surplus of RE electricity, and to further develop interseasonal heat storage[7].
The efficiency of P2H coupling via district heating is enhanced by the storage potential of district heating systems, as Denmark is starting to experiment, both for the short term and for interseasonal use[8]. In the latter case, the energy produced in the form of electricity by wind turbines in the autumn, a period of high production, is consumed as heat in the winter. This allows P2H to track hourly electricity price changes, while the district heating network can decouple the production and demand for heat from the district heating network during peak periods. The development of storage leads to postponing investments in new boilers. It is also the way to indirectly increase the supply of RE to district heating systems without additional investment in biomass boilers (Figure 3).
In a well-integrated energy system, a district heating supplier can react to price fluctuations on the electricity market and contribute to the balance of the network by producing or consuming more electricity. Cogeneration allows some form of thermal storage, which provides flexibility for combined heat and power systems. District heating companies can also use thermal energy storage, which is generally cheaper than electricity storage, to give greater flexibility to the overall system (Read: Co-generation and seasonal heat storage).
District heating is ideal for P2H sector coupling. Two ways of sector coupling are possible:
- combine heat pumps and boilers based on renewable energy that operate in a complementary manner, the former during periods of low electricity prices resulting from hourly surpluses of RE electricity, the latter during periods of high prices ;
- a combination of heat pumps and cogeneration that can easily switch to heat-only mode during low electricity price episodes, allowing a form of thermal storage and giving flexibility to electricity, heating and gas systems.
District heating systems then offer several types of flexibility (Figure 4):
- p2H technologies can absorb wind generation peaks to meet heat demand and store excess electricity available at low cost as heat through electrical energy conversion (Case 1 in the figure) ;
- biomass boilers and thermal storage can produce heat during periods when no additional flexibility is needed in the electricity market (Case 2 in the figure);
- cogeneration plants can meet electricity demand when wholesale electricity prices are high during low wind generation episodes (Case 3 in the figure).
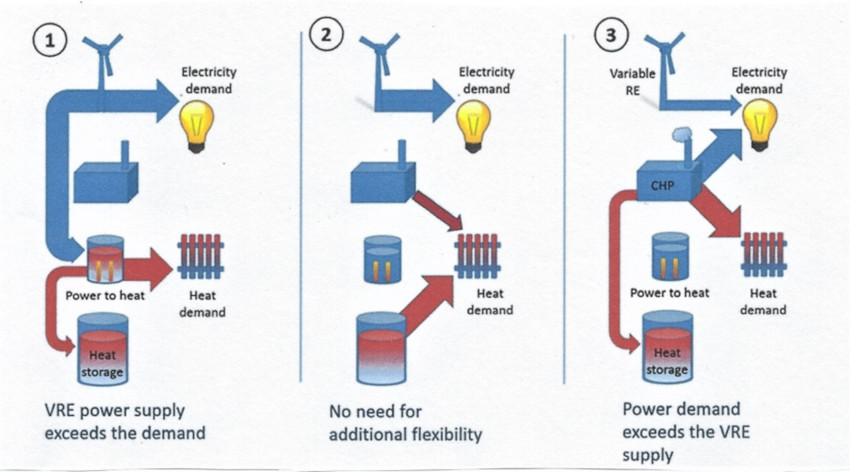
Fig. 4: The different forms of flexibility offered by the P2H coupling based on a district heating system. [Source: © Skytte et al. 2019, Flexible Nordic Energy Systems, DTU Library. Acronym: VRE = Variable Renewables Electricity]
In France, a study on heat storage and P2H potentials has estimated the development potential of heat pumps on district heating networks at nearly 1,000 MW by 2030, excluding geothermal energy[9]. This solution constitutes an alternative to the use of waste and biomass, while making it possible to reduce natural gas consumption during semi-peak demand periods. However, the value of heat pumps and their deployment will be strongly linked to the evolution of temperature levels on the networks.
To sum up, in district heating networks, but this may also be the case for heating large tertiary buildings, heat pumps coupled with networks supplied by cogeneration plants powered by renewable energies, offer significant possibilities for decarbonising heat uses. However, strategies should be chosen taking into account the other possibilities for district heating networks to use other renewable energy sources such as geothermal, biomass, industrial waste heat and solar thermal energy. As electricity generation increasingly relies on RE, the flexibility offered by this sectoral coupling is of increasing economic value. Thus, Denmark, with its combination of large district heating networks and a very large wind energy capacity, has a strong case for developing P2H cross-sectoral coupling[10].
3. The place of Power to Gas
P2G is based on electrolysis processes, including the alkaline process that is currently the most economically feasible, extended by the production of synthetic natural gas through methanation. All of the electrolysis processes must all advance to eventually compete with the conventionalCO2-emitting natural gas steam-reforming process. Experimental pilots of P2G, intended to compete with the conventional process, were developed in the late 1990s and early 2000s, but with no promise of possible commercial development[11].
Those who advocate the idea of setting up a 100% RE power system even propose to produce electricity again from this synthetic methane at the right times. This project to make P2G a means of inter-seasonal storage ignores the low efficiency of successive operations, i.e. 55% forH2 and GNS production, or 25% for the gas turbine that succeeds it, hence the high cost of the MWh that would have been stored in this way. It is on this idea that Power to Gas was first put on the front of the stage because, for some engineers, it is the means to completely loop an electrical system entirely based on RE, while offering the only possibility of interseasonal electrical storage[12].
At the same time, the desire to replace natural gas by green gas in the gas system should lead to building bridges between this system and electricity production, with hydrogen production by electrolysis and GNS (Read: Hydrogen). The Power to Gas sectors are of interest to the players in the gas system who need to perpetuate the current uses of natural gas by highlighting the possibilities of its complete decarbonisation through the gradual greening of the gas system, following the example of what we are trying to do with the electricity system, because this is the only way to hope to have large quantities of decarbonated gas, with extensions in the use of synthetic natural gas for transport. This is also the way in which the Power to Gas sectors could take shape as part of the greening of the gas system and the development of a hydrogen economy directed towards different types ofH2 use in industry, transport and the gas system (Figure 5).
Focusing only on the transformation of the carrier, from electricity to hydrogen and then to methane, as P2G advocates do, leads to ignoring the difficulties of transforming the gas system into a system based solely on green gases (biogas, biomethane,H2 from P2G, synthetic methane). This criticism also applies to the implementation of an all-hydrogen economy, of which P2G could be the keystone, by combining it with the electricity economy with a very high share of EnRi. Going further, P2G advocates present the development of P2G processes as a means of adding value to additional EnRi’s electrical capabilities to further develop their place in the overall energy system. But to achieve this, the obstacles to be overcome are considerable: difficulties in installing equipment with very high capital expenditure (capital expenditure -CAPEX) in an environment of very uncertain prices; in the case of the hydrogen economy, the need to set up a new transmission and distribution infrastructure subject to strict safety constraints
In order to fully understand the scope of these obstacles, it is essential to restart gas production from RE electricity, to question its possible contribution to the greening of the gas system and to assess the constraints to the development of a hydrogen economy in which green electricity production or, more broadly, low-carbon electricity production (RE and nuclear) would be integrated.
3.1. The place of P2G in the greening of the gas system
In any case, green hydrogen and the methane resulting from its methanation will compete with other green gases, biogas and biomethane, in policies to replace fossil natural gas[13] (Read: Gasification). What are biogas and biomethane about?
Resulting from the anaerobic digestion of various biomasses, biogas consists of 50-65% methane, sometimes up to 50% carbon dioxide (CO2) and small amounts of other gases and impurities. Most biogases are used locally near their point of production to obtain electricity, heat, or both. Each production facility is small, between 1 and 2 MW, and biomethane, which is more relevant than biogas to play an important role in the desired transformation of the gas industry, typically contains more than 90% methane. It can therefore be injected into the gas network where it can be mixed with fossil natural gas (Read: Methanisation: from wastewater treatment to injecting biomethane into the network). It is produced almost exclusively from biogas produced by anaerobic digestion with separation of methane fromCO2
and other impurities to obtain an acceptable composition for the natural gas network, which can be done by various methods: absorption, membrane filtration or cryogenic separation. By 2016, its production had reached 17 TWh in Europe, equivalent to approximately 1.6 billion cubic metres (bcm) of natural gas, or less than 1% of total natural gas consumption.
In a normative prospective for the total greening of the French gas system by 2050, the French Environment and Energy Management Agency (ADEME) published four scenarios in 2018. The agency considers P2G in only one of them, but in all of them it identifies two other technologies for developing biomethane on a large scale: methane production from organic matter through anaerobic digestion and pyro-gasification, which produces methane through the thermochemical transformation of ligno-cellulosic resources. These options would undoubtedly provide access to a larger biomass potential, but they are not based on any economic analysis, however serious.
Biomethane production will therefore always remain limited by the availability of suitable raw materials, whereas the use of P2G is limited only by the availability of electricity at low hourly prices, which conditions the rate of use of equipment and the profitability of investments in electrolysis and methanation[14].” If low-cost electricity (<€15/MWh) is available 75% of the time, the cost of methane from P2G could approach €50/MWh, which is in line with current and recent natural gas market prices of €20 to €40/MWh. On the other hand, if low-cost electricity is available only 10% of the time, with an average annual electricity price of around €40-50/MWh, the cost of gas could reach €170/MWh”[15]. This is confirmed by the ENEA baseline study “The potential of Power to Gas“, which finds a cost price for synthetic methane in 2030 of €170 to €185/MWh, significantly above the price of fossil natural gas, which is around €20/MWh in 2020[16]. It is necessary to wait for significant reductions in the cost of technologies and hourly electricity prices (made possible by the development of RE on a very large scale) to reach €95/MWh in 2050.
A major unknown regarding the large-scale implementation of P2G remains: the choice of the H2 transmission infrastructure, as it is a key element of its economic future. Indeed, when one wants to go beyond the stage of using H2 produced in P2G by mixing it up to 5% with fossil natural gas, one encounters the alternative of converting the current infrastructure from methane to hydrogen, or converting H2 to methane and then transporting this green methane into the current gas system. In the first case, the transformation of the infrastructure is costly with the upgrading to specific safety standards, bearing in mind that this transformation also involves the transformation of equipment currently using the gas for heat production. In the second case, the transformation of RE electricity into hydrogen must be complemented by its methanation into GNS.
3.2. P2G in the development of a hydrogen economy
The development of a hydrogen economy refers to ideal scenarios in which hydrogen is assumed to become the second basic vector for decarbonation of the energy system. This vocation must impose it despite the risks of some trajectory blockages. For production, electrolysis must take precedence over the conventional steam-reforming production process. It must be powered by low-carbon electricity, mainly of renewable energy origin via the P2G gateways.
3.2.1. The various possible uses of hydrogen
The large-scale development of hydrogen as a clean energy carrier is not a new idea, but there is no consensus on how best to deploy the hydrogen economy in a decarbonized energy system.
- A first possibility is a direct use as a transport fuel, through the use of fuel cells installed in electric motor vehicles, but this type of vehicle will compete with the battery electric vehicle, which should limit its development in the light vehicle niche (Read : Fuel cells).
- Hydrogen could also be used industrially in current uses of H2 – a raw material, but green H2 would then compete with H2 produced by steam reforming of natural gas.
- Another industrial use would be the production of heat, in industrial applications that require high temperatures .
- Hydrogen can also be injected directly into methane networks, up to a few percent under strict safety standards. Too high a hydrogen content would raise technical and safety issues. A content of up to 5% would be acceptable without affecting the gas network or end-user equipment[17]. Such an injection would save network infrastructure to disseminate this new green gas, but this solution is clearly limited. On the other hand, hydrogen can also be injected indirectly into the system after it has been transformed into methane, by combiningH2 withCO2, particularly from carbon dioxide capture.
3.2.2. The foundations of a hydrogen economy
The development of an integrated hydrogen economy that would gradually replace the current gas economy requires significant investment in large-scale H2 production, storage, transport and distribution at filling stations under strong safety constraints. In transport applications for which delivery by truck would be sufficient, it is necessary to ensure the safety of depots and service stations with pressurized hydrogen tanks, including those located in the heart of cities.
Economic barriers will arise on several levels. Firstly, the profitability of equipment for the production ofH2 by electrolysis, which has a high CAPEX, is exposed to many risks, particularly those linked to the uncertainties of electricity price levels and their evolution. Secondly, the development of transport infrastructures requires very significant investments and good coordination with the development of consumption in the different types of use.
At these different levels, competition from other energy carriers, including fossil natural gas, will be a decisive obstacle. This is particularly the case for competition between fuel cell vehicles and battery-powered electric vehicles[18], the former being constrained by the development of a sufficient number of specialised service stations and the latter by the existence of a network of recharging stations. The current consensus of experts only gives H2 and fuel cells an advantage in long-distance heavy transport, by rail and sea, or in certain intra-urban heavy transport, particularly refuse collection.
3.2.3. An opportunity for decentralised development
The development of green hydrogen in industrial uses, namelyH2-fueland high-temperature heat uses, can be exempted from the development of networks because production by electrolysis can be done in situ, on industrial sites. The uses on each site may be large enough to allow the installation of large electrolysers with up to 10 MWe of operating power, which will be cheaper than the current electrolysers, which are mostly 1 MWe.
The development of complete hydrogen systems including P2G can also be carried out at the local level of a city, as South Korea is experimenting in three new city projects[19], which would avoid transforming the entire pipeline system or developing a new specific transport system. In this approach, the city develops, with financial support from the State, production equipment (electrolysers), network and service station infrastructure, fuel cell vehicle fleets and the installation of large fuel cells in the commercial and tertiary sectors. Ideally, the majority of electricity comes from ground-based solar PV installations or wind turbines installed nearby in a local version of P2G. If this pattern were to become widespread, we would be faced with a low-carbon transition developed from the local level. History will tell whether this type of project is likely to trigger a dynamic of development of the hydrogen economy from below. For this to happen, this option will have to prove to be economical compared to the centralised fossil natural gas system.
4. Inter-sectoral Electricity-Transport Couplings
In transport, the coupling of RE-based electricity production and transport demand should be the main axis of greening the sector, via the electrification of the transport sector (Read: The car of the future: techniques in competition). Synthetic natural gas for vehicles (CNG), produced from the methanation of green hydrogen, and biofuels, which should complement this greening, are possible options, but they are expensive and bring little in terms of carbon footprint, even when biofuels are third generation.
4.1. Electromobility, a major bridge between sectors
The decarbonisation of transport is a challenge that can only be met by the large-scale adoption of very low-emission vehicles powered by low-carbon energy carriers, which will mainly be achieved through electromobility (e-mobility) consisting of battery-powered electric vehicles (EVs) and fuel cell EVs, the former facing fewer development constraints than the latter (specific transport infrastructure, heavy logistics service stations, high safety constraints). However, some life-cycle analyses reveal that segments of the mobility market, particularly those of captive fleets and certain modes of freight transport, could be better served by hydrogen or synthetic methane than by batteries. In other words, for long-distance transport, in particular road transport, shipping and aviation, a combination of hydrogen vehicles and hydrogen-derived synthetic liquid fuel vehicles could be an appropriate solution.
4.2. interactions between the electric and transport sectors via EV batteries
An interest in the development of electric mobility is the possibility offered by the integration of EVs and their batteries into the electrical system for its balancing and stability through the electrical storage capacity that the EV fleet will offer. It is indeed likely that in the coming years, the development of this storage mode will dominate due to the deployment of EVs and the difficulties of making battery or compressed air storage projects profitable, not to mention the saturation of hydraulic pumping sites[20].
However, before this promise can be realized, the impact on the EV battery recharging system will first have to be minimized by placing EV batteries in such a way as to avoid the evening peak in favour of night charging, which can be achieved on the well-accepted model of night-time powering of electric water heaters. This type of extraction control, known as V1G (Vehicle to Griden, simple mode), has every chance of finding its economic model and developing rapidly.
There is also talk of V2G, which is the reverse use of EV batteries for balancing and stability of the electrical system[21]. In an idealized vision, operators would aggregate batteries in large cohorts to inject power to support the power system during drops in EnRi production. This Vehicle to Griden payout would operate on the basis of compensation to vehicle owners. However, while the economic interest of V1G control is proven and the solutions available, V2G requires the development of a specific digital infrastructure and raises questions :
- this can be done using technical methods, such as battery charging,
- economic, such as technical losses or compensation to owners for the service to be valued,
- such as the type of contracts.
The future of the V2G therefore seems much less certain than that of the V1G.
See 2nd part of the article ( Power to Gas and Power to Heat Cross-Sectoral Couplings: What Role in the Energy Transition? (2nd part)) which also includes full bibliographic references and two annexes:
- P2G processes for the production of “electro-green” gases
- The economic evaluation of Power-to-Gaschannels
References
Cover image. [Source: Natural Gas Generator]
[1] The bibliography of this article as well as the appendix entitled “P2G processes for the production of electro-green gases” are published at the end of Part2of this article.
[2] The acronyms PtoH and PtoG are also used
[3] District heating can use multiple renewable energy sources such as low-enthalpy geothermal, solar thermal and biomass sources including household waste. It can also use waste heat from power plants or industrial processes, and solar thermal.
[4] Note that heat pumps are theoretically reversible and would therefore also be able to supply cold to buildings. Of the 800,000 heat pumps sold in Europe in 2015, more than 450,000 were reversible.
[5] Kiss, Nedj, Jakob, 2011
[6] This is discussed below.
[7] Bergaentzle et al, 2018.
[8] Short-term storage refers to hourly and daily storage in hot water tanks as well as in the district heating system itself. Seasonal heat storage refers to thermal storage between seasons, often with a slower rate of filling and emptying than for short-term storage techniques, but with the possibility of storing thermal energy for longer periods.
[9] ADEME, ATEE, Etude PEPS3, 2016.
[10] Skytte et al. 2018.
[11]Annex 1 (Part2 ) presents the three electrolysis processes developed or under development, as well as the two greenH2 methanation processes, catalytic methanation and biological methanation
[12] See the first ADEME study on 100% electric RE (ADEME, 2016)
[13] Lambert, 2018.
[14] The number of hours when electricity market prices are low depends closely on the share of EnRi in electricity generation.
[15] Lambert, 2018, pp. 5-6.
[16] ENEA study, 2016, summarized in Annex 2 (Part2).
[17] The European Commission is in the process of normalizing the percentage of contents so as not to block gas exchanges between countries.
[18] Lesser, 2019.
[19] See Eric Lesser’s article of 10 December 2020, https://www.transitionsenergies.com/coree-sud-villes-nouvelles-hydrogene/
[20] One million battery-powered vehicles will account for 40 to 70 GWh of energy storage capacity/day, or a demand of around 10 GWh of daily recharges. This corresponds at most to a grid demand of 1500 to 2500 MW if all the recharges of the day are done at the same time, based on an average recharge power of 10 kW per battery.
[21] Merley and Vilain, 2019.
The Energy Encyclopedia is published by the Association des encyclopédies de l’environnement et de l’énergie (A3E)(www.a3e.fr), contractually linked to the University of Grenoble Alpes and Grenoble INP, and sponsored by the French Academy of Sciences.
To cite this article, please mention the name of the author, the title of the article and its URL on the Encyclopedia of Energy website.
The articles in the Energy Encyclopedia are made available under the terms of the Creative Commons Attribution-Noncommercial-No Derivative Works 4.0 International License.